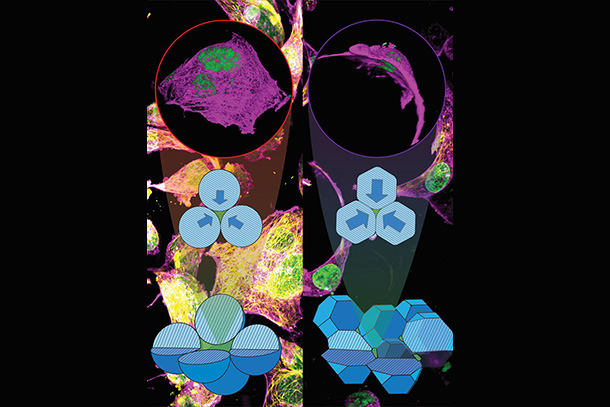
Researchers have discovered that by adjusting how gelatin-based hydrogel microparticles, which are tiny gel-like particles, are arranged in scaffolds, they can control the shape and movement of cells. In lab settings, cells can switch from a spread-out form to an elongated shape, and in living tissues, the process influences how cells move into the scaffold. This could be important for developing consistent methods to create these hydrogel scaffolds for both lab-grown tissues and treatments that help repair damaged tissues inside the body, the researchers said. Credit: Amir Sheikhi.
Squishy microgels in granular biomaterials confine and direct cell behavior
The work, conducted in mice, may have implications for more effective medical therapies to heal wounds, treat cancers or regenerate organs by regulating cell behavior, the researchers said
Nov 13, 2024
Editor's note: This article originally appeared on Penn State News.
By Jamie Oberdick
UNIVERSITY PARK, Pa. — A simple biomaterial-based strategy that can influence the behavior of cells could pave the way for more effective medical treatments such as wound healing, cancer therapy and even organ regeneration, according to a research team at Penn State.
The team’s work, published in Advanced Healthcare Materials, and featured as the frontispiece, focused on manipulating support structures made of soft, squishy, water-absorbing microscale materials to create customizable scaffolds that partially mimic the physical properties of natural mammalian tissues. Called gelatin methacryloyl (GelMA) granular hydrogel scaffolds, the materials comprise hydrogel microparticles known as microgels that can be squeezed against each other and confine cells among them at varying extents.
“We’ve found that by adjusting the packing density of microgels within the granular hydrogel scaffolds, we can control how cells behave,” said corresponding author Amir Sheikhi, associate professor of chemical engineering and the Dorothy Foehr Huck and J. Lloyd Huck Early Career Chair in Biomaterials and Regenerative Engineering. “This allows us to create a microenvironment that directs cell behavior in ways we couldn’t achieve before.”
The ability to manipulate the structure of hydrogels in this way would enable scientists to design medical treatments tailored to the unique needs of different tissues and healing processes.
Hydrogel scaffolds have long been used in medical research and treatments such as supporting blood vessel formation and direction during reconstructive surgery, but Sheikhi said the level of control he and his team were able to exert over the internal structure of the hydrogel is exciting. By packing the microgels less densely within the scaffold, the researchers found they could create environments that promote cell migration and proliferation.
“Loosely packed scaffolds create larger pores that are ideal for promoting cell infiltration and tissue integration,” Sheikhi said. “This freer cell movement could promote wound healing and integration of new tissue into the body.”
Conversely, the researchers found that the smaller pores of densely packed scaffolds cause cells to adopt a more confined, elongated shape and restricts locomotion. This kind of control over cell behavior could be especially useful in modeling confined, solid tissues and testing treatments to inhibit cell migration such as modeling the cancer tumor microenvironment modeling and and testing how to prevent metastasis, according to Sheikhi.
For wound healing, Sheikhi said the research team envisions designing scaffolds that are custom-made to promote the best recovery environment via cell recruitment for specific tissues.
“By varying the packing density of GelMA microgels, we can directly influence the mechanical properties and pore structure of the granular hydrogel scaffold,” Sheikhi said. “This allows us to create scaffolds that are tailored to the specific needs of different tissues, ultimately leading to more effective treatments.”
Not only could the scaffolds be tailored to specific tissues, they could also potentially be customized for individual people.
“We propose a future where we can create scaffolds that are custom designed for individual patients, providing more personalized and effective treatments,” Sheikhi said. “This could not only improve patient outcomes but also reduce recovery times and complications, making health care more efficient.”
More broadly speaking, these hydrogel-developed tissue models could also improve how diseases are studied and treated, according to Sheikhi. The ability to create hydrogel scaffolds with varying mechanical properties, decoupled from the local stiffness, could lead to more accurate models of human tissues, which in turn would enable scientists to better study how diseases like cancer progress. These disease models could also be used to test new drugs in a laboratory setting before they are introduced into clinical trials.
“We’re excited about the potential for using these granular hydrogel scaffolds in the development of tissue and disease models on a chip,” Sheikhi said. “These models could provide researchers with more reliable platforms for testing treatments and understanding disease behavior, speeding up the discovery of new therapies.”
Next, Sheikhi said the team plans to test how the materials work with human tissue and to investigate scaling-up production of the hydrogel scaffolds while maintaining consistent quality.
“Addressing these challenges will be crucial for successfully bringing these innovations from the lab to the clinic,” Sheikhi said.
Along with Sheikhi, other authors of the study include Arian Jaberi, doctoral candidate in chemical engineering; Alexander Kedzierski, graduate research assistant in biomedical engineering; Sina Kheirabadi, doctoral candidate in chemical engineering; Yerbol Tagay, doctoral candidate in the College of Medicine; Zaman Ataie, doctoral candidate in chemical engineering; Saman Zavari, doctoral candidate in chemical engineering; Mohammad Naghashnejad, postdoctoral scholar, chemical engineering; Olivia Waldron, medical doctor candidate in the College of Medicine; Daksh Adhikari, undergraduate research assistant in chemical engineering; Gerald Lester, undergraduate research assistant, chemical engineering; Colin Gallagher, undergraduate research assistant, chemical engineering; Ali Borhan, professor of chemical engineering; Dino Ravnic, Dorothy Foehr Huck and J. Lloyd Huck Chair in Regenerative Medicine and Surgical Sciences and associate professor of surgery; and Erdem Tabdanov, assistant professor of pharmacology.
The National Institutes of Health, Huck Innovative and Transformational Seed Fund, Materials Research Institute Seed Grant for Convergent Research at the Intersection of Materials-Life-Health-Environment and College of Engineering Materials Matter at the Human Level seed grant supported this work.